“Think about drops of water in their natural context, which is in the clouds,” says Himanshu Mishra, an expert in water interfaces. “If you do a rough calculation, the surface area of all those droplets is close to 50 times that of the planet itself. Any chemical reaction involving water surfaces can become quite significant on these droplets; much more than on the ocean surface, for example.”
In 2019, Mishra became aware of a new study that could upend conventional understanding of water surfaces. A team from Stanford University reported that fine sprays of water, containing droplets no more than 20 micrometers wide, could spontaneously generate hydrogen peroxide — an energetically unfavorable chemical reaction that normally requires catalysts to be successful.
The Stanford team postulated that ultrahigh electric fields at the microdroplet interface could pry electrons away from water molecules, leading to peroxide formation. But to Mishra, this explanation failed to add up.
“Why would the surface of water do that?” asks Mishra. “If it has something to do with the polarity of the oxygen-hydrogen bond, then you would imagine the same features should appear in methanol and other alcohols.”
From previous experience studying water sprays, Mishra knew that such microdroplets needed to be characterized with a combination of techniques to eliminate the possibility of artifacts influencing the experimental results. For this task, he enlisted three of his team members: Adair Gallo Jr., a specialist in generating sprays, and Nayara Musskopf and Peng Zhang, who established protocols for detecting ultra small quantities of hydrogen peroxide.
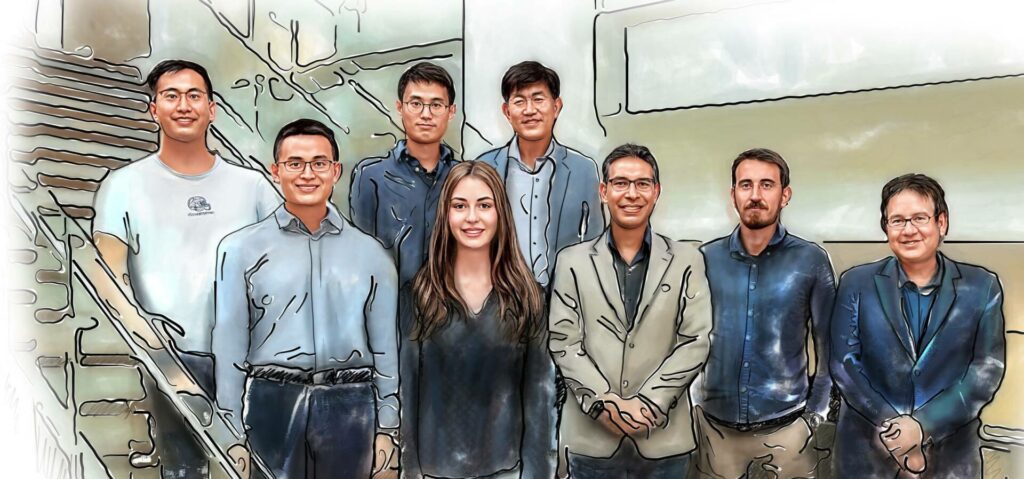
“We couldn’t detect hydrogen peroxide at the concentrations they were reporting, and we tried many different types of spraying conditions,” says Gallo Jr. “We even used a fluorescence kit with about 40 times the detection sensitivity, but we still couldn’t see it,” adds Musskopf.
Next, the team turned to their colleagues at KAUST to help sort out the peroxide puzzle. “We thought that it might have something to do with the shockwaves generated by the sprays,” recalls Mishra. “We requested mechanical engineer Hong Im and his team to carry out simulations of the problem and, with fellow mechanical engineer Sigurdur Thoroddsen and his team, we tried to examine the microdroplets with high-speed imaging.”
“We thought that it might have something to do with the shockwaves generated by the sprays”
Even with the extra brainpower on the case, the researchers could not find evidence of hydrogen peroxide. “There were some shockwaves,” says Mishra, “but those conditions were pretty mild and not strong enough to drive spontaneous chemistry. Siggy (Sigurdur Thoroddsen) and Hong helped us nail down some null results to our story.”
After a year of experiments that seemed to go nowhere, even the most dedicated researchers would begin to have doubts. “I think I resigned from the project at least twice,” admits Gallo Jr. “We really didn’t want to face this anymore.”
Eventually, serendipity came in the form of a 40-year-old article about atmospheric chemistry. While studying the paper, Mishra came across a paragraph mentioning artifacts that can influence peroxide studies — one of them being ozone molecules.
“When I read this, I just jumped and ran to my lab to tell Adair. We have an ozone generator, we turned it on with the water microdroplets, and for the first time, our peroxide detection strip changed color,” remarks Mishra. “We deduced that there is just more ozone in the Stanford lab, and in the air in California, because of pollution.”
Recently, the Stanford researchers admitted that ozone may be behind the anomalous generation of hydrogen peroxide signals and are now taking great care to eliminate the molecule from microdroplet experiments.
“When you’re claiming something about water that’s almost like alchemy, you really have to drill down and check with multiple techniques before changing the chemistry textbooks,” says Mishra.